

Proteins & Origami Research
How similar are proteins and origami ?
Answering this question may contribute to our understanding of proteins, how they fold, and how protein structures contributes to the function it fulfills.
Comparing protein structures and origami models
We proposed a new approach for the comparing of protein structures with origami models. In the process, a protein of interest is selected, and analog origami model/s are identified. Based on the features of the analog origami models and the function that the protein performs, the structural characteristics of the protein are analyzed.
As a proof of concept, a comparison and analysis of OmpA β-barrel and α-helical TM barrel of the MscL with the Yoshimura and Kresling origami models is performed (Picture on the right). The function that the proteins fulfills as well as the unique mechanical characteristics of the origami models, shed light on the way that these protein structures are designed to fulfill their function.
Based on the analogy and the characteristics of the Kresling model, it is suggested that these protein structures are specifically designed to adjust diameter and height by changing the β-strands/α-helices tilt in response to axial, radial or twist loads from changes in the lipid of the membrane.
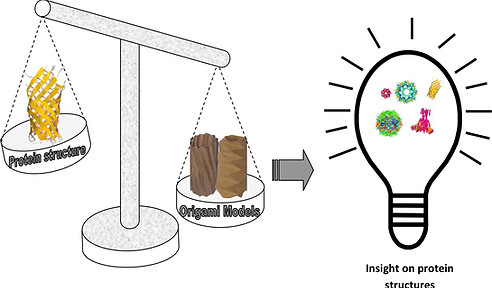
Reprint with permision from Elsevier
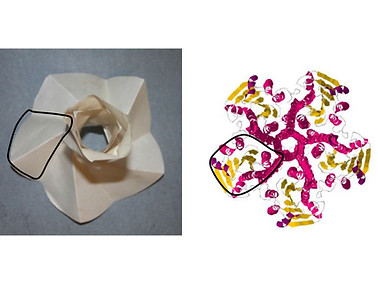
Reprint with permision from Elsevier
Comparing mutli-domain protein structures and origami models
We applied the approch for comparing protein structures and origami models to study multi-domain proteins. For example, it is shown that the the CorA protein is similar to a newly folded origami model that combines the Kresling and Flasher origami models (picture on the left). Based on the similarity and the charactaristics of the the origami models, it is proposed that the CorA structure is auxetic.
How Similar are Proteins and Origami ?
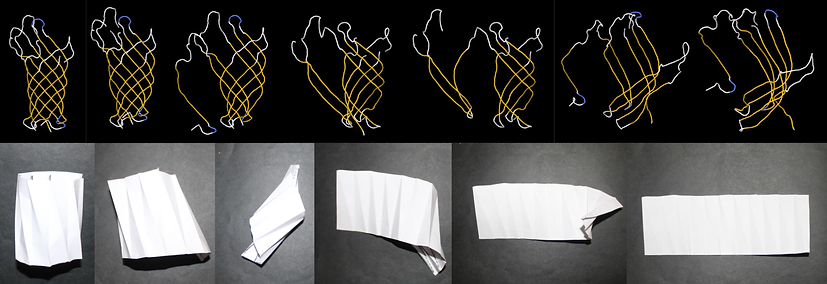
Reprint with permision from MDPI
In this paper we studied the equivalence of proteins and origami. Research on proteins and origami provides strong evidence to support the use of origami folding principles and mechanical models to explain aspects of proteins formation and function. Although not identical, the equivalence of origami and proteins emerges in: (i) the folding processes, (ii) the shape and structure of proteins and origami models, and (iii) the intrinsic mechanical properties of the folded structures/models, which allows them to synchronically fold/unfold and effectively distribute forces to the whole structure. As a result, origami can contribute to the understanding of various key protein-related mechanisms and support the design of de novo proteins and nanomaterials
Studying Biomolecular Complexes via Origami and 3D-Printed models
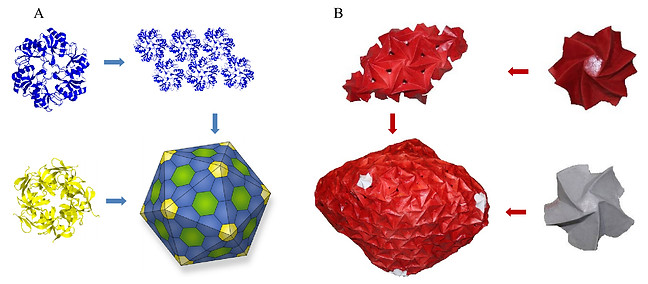
Reprint with permision from MDPI
Living organisms are constructed from proteins that assemble into biomolecular complexes, each with a unique shape and function. Our knowledge about the structure–activity relationship of these complexes is still limited, mainly because of their small size, complex structure, fast processes, and changing environment. Furthermore, the constraints of current microscopic tools and the difficulty in applying molecular dynamic simulations to capture the dynamic response of biomolecular complexes and long-term phenomena call for new supplementary tools and approaches that can help bridge this gap. In this paper, we present an approach to comparing biomolecular and origami hierarchical structures and apply it to comparing bacterial microcompartments (BMCs) with spiral-based origami models. Our first analysis compares proteins that assemble the BMC with an origami model called “flasher”, which is the unit cell of an assembled origami model. Then, the BMC structure is compared with the assembled origami model and based on the similarity, a physical scaled-up origami model, which is analogous to the BMC, is constructed. The origami model is translated into a computer-aided design model and manufactured via 3D-printing technology. Finite element analysis and physical experiments of the origami model and 3D-printed parts reveal trends in the mechanical response of the icosahedron, which is constructed from tiled-chiral elements. The chiral elements rotate as the icosahedron expands and we deduce that it allows the BMC to open gates for transmembrane passage of materials.